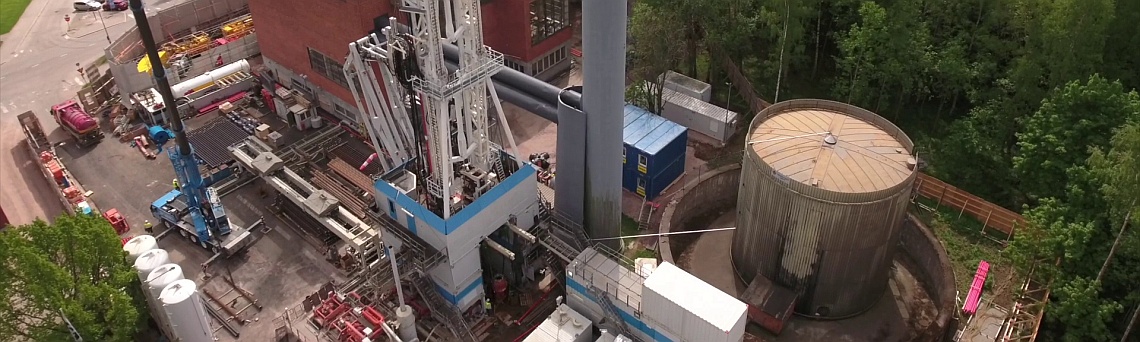
St1 Deep Heat project
The St1 Deep Heat project site is located in Helsinki suburbian area at the Aalto University in Espoo, approximately 6 km away from Helsinki city center. The project aims to provide a sustainable baseload for the campus area heating network by extracting the hot water from the depth of over 6 km. The intended configuration is to have a well doublet, where one well will be used for (cold) water injection and second well for (hot) water extraction. In the first stage of the drilling, a 6.4 km measured length well OTN-3 was drilled, with the last 1000 meters of the inclined open-hole section. The last part of the well targeted permeable geological formations at 5-6 km depth with temperatures up to 120C.
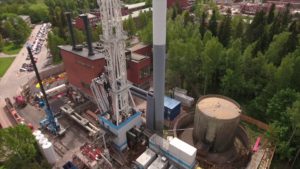
The designed Enhanced geothermal systems (EGS) hold the promise of using the ubiquitous heat energy of Earth. However, enhanced geothermal systems typically requires opening – so-called “stimulation” – of fluid flow channels to enhance the permeability of the new reservoir. The natural by-products of this engineered process are earthquakes. Seismic activity related to enhanced geothermal systems have seriously affected or even terminated some geothermal projects such as Basel, Switzerland in 2006 or Pohang, South Korea in 2018. Therefore, implementing of safe stimulation strategies was critical for public acceptance of designed enhanced geothermal system.
Before this could happen, it was necessary to hydraulically stimulate the future geothermal reservoir by injecting fluids into the OTN-3 well in order to enhance its permeability. The stimulation was performed in Summer 2018. In the following stage, a new well will be drilled into the created damage zone in order to establish the well doublet and to start the commercial exploitation of the heat.
Preparation for reservoir stimulation
The stimulation monitoring network, designed by ASIR LLC, was composed of 12 sensors located at depth 2.6 km in adjacent borehole OTN-2, approximately 3 km above the injection area. This was completed by additional 12 borehole sensors located up to 11 km away from the project site. A separate seismic network of 17 geophones was installed in locations critical from perspective of ground motions, and it was used by ARUP Geohazards to support the traffic light system operations.
2018 stimulation campaign
Near-realtime monitoring system
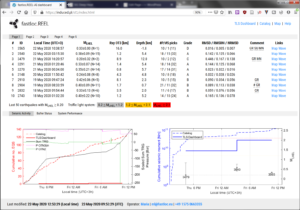
For near-realtime seismic monitoring and reporting, the fastloc.REEL system was used, which I developed for fastloc GmbH. This system detected and located more than 8,000 earthquakes in the vicinity of project site during the stimulation campaign and shortly afterwards. The resulting first-break information included initial hypocenter location and magnitude estimate which were both available up to 5 minutes after the earthquake occurrence. In case of larger events, the magnitude estimate and hypocenter location was manually verified by the seismologist from fastloc GmbH team working remotely and on-site. The catalog of seismic events was updated continuously during injection operations and provided information both to the Traffic Light System (TLS) operator as well as injection engineers, both located at the project site in Espoo. All parties were informed through three independent channels: 1) The dedicated website (“dashboard”), where catalog of seismicity and its evolution was presented in near-real time, 2) the SMS-like pushover alert system, and 3) email alert messages.
During the stimulation, the seismicity evolution was used to decide in ample time on how to change stimulation parameters. Our fastloc GmbH team also served their expertise while discussing the modifications in injection program in response to the development of induced seismicity together with other partners. By the end of the project at 18,500 m3 of fresh water injected, the maximum observed local magnitude was ML 1.9, which was just below the red alert level of ML 2.1 set up by the local authorities.
Controlling induced seismicity
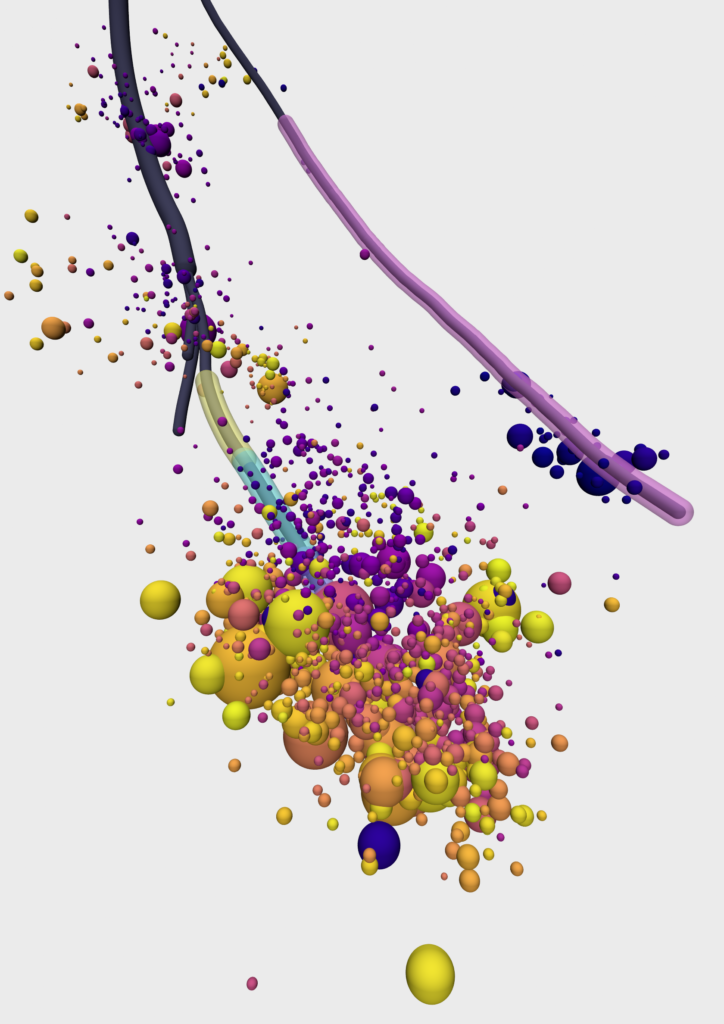
Already at the begin of stimulation (Phase 1-2), the project team quickly realized that the seismic energy release is proportional to the hydraulic energy, or equivalently product of pressure and volume. It was also identified that any stop in injection leads to quick reduction in seismic activity. In Phase 2 of injection, the maximum well head pressure of around 90 MPa was applied, and the injection was performed with long intervals lasting up to 3 days. This resulted in acceleration of seismic energy release and led ultimately to a series of relatively “amber” earthquakes that put the injection temporarily on hold. It was obvious for the project team that a redefinition of the injection strategy is required.
The project team realized that the maximum observable magnitude increase with cumulative injected fluid volume. Surprisingly to the team, the trend of this magnitude evolution with injection already seemed to nicely follow that predicted from the fracture mechanics-based of Galis et al. (2017). While extending the observed trend to the planned total volume of 20,000m3, it was a concern of project team that by the end of the red alert ML 2.1 event may occur.
Galis model relates maximum magnitude of the so-called arrested rupture to the amount of energy available stored for rupture propagation. The project team decided to reduce somehow the amount of hydraulic energy stored in the geothermal system. At first, the well head pressure was reduced to values below 90 MPa in attempt to reduce the hydraulic input energy rate into the developed geothermal system in Phases 3 of injection. In following phases 4 and 5 injection plan was progressively changed. The injection was still performed at lower injection pressure, but the injection times were progressively reduced and the resting periods in-between stimulations were enhanced. This strategy visibly stabilized the seismic energy release with respect to hydraulic energy input. Till the end of the project, the red alert threshold was not exceeded, as the maximum observed magnitude reached M1.9 in the stimulation stage 4.
2020 Stimulation Campaing
Between May 4t, 2020 and May 20th, 2020, 2875 m3 of water has been injected into the open-hole section spanning between depths 4856-5765 m of the deepened OTN-2 well, to establish the communication between OTN-2 and OTN-3 wells. Similarly to 2018 stimulation campaign in OTN-3, the 2020 stimulation was injection-rate controlled. During injection, a total of 2,875 m3 of fresh water was injected during 16 days at well-head pressures <70 MPa and with flow rates between 400-1000 l/min. This led to occurrence of much smaller levels of induced seismicity related to proportionally lower amount of hydraulic energy “injected” into the geothermal system. A total of 6,121 earthquakes indicating fractures of 1-30m size were recorded during and after stimulation campaign.
Using a handful of statistical properties derived from earthquake catalog we found no indication for earthquakes being triggered by other earthquakes. Instead, the earthquake activity rates, as well as the maximum earthquake size stayed proportional to the fluid injection rate. The spatio-temporal behavior of seismicity and its properties suggest earthquakes occurred not on a single fault, but in a distributed network of similarly oriented fractures, limiting the possibility for occurrence of violent earthquakes. The performed study provided evidence that the induced seismicity due to injection performed within St1 Deep Heat project is stable and allow to constrain seismic hazard.
Publications
Our team at Section 4.2: Geomechanics and Scientific Drilling of GFZ German Research Centre for Geosciences is continuously working on seismic data from both stimulation campaigns, together with industrial and university partners participating in the St1 Deep Heat project. So far these papers has been published related to the project:
- Kwiatek, G., T. Saarno, T. Ader, F. Bluemle, M. Bohnhoff, M. Chendorain, G. Dresen, P. Heikkinen, I. Kukkonen, P. Leary, M. Leonhardt, P. Malin, P. Martínez-Garzón, K. Passmore, P. Passmore, S. Valenzuela, and C. Wollin (2019). Controlling fluid-induced seismicity during a 6.1-km-deep geothermal stimulation in Finland, Sci Adv 5, no. 5, eaav7224, DOI: 10.1126/sciadv.aav7224. [ Article Page ] [ Download open-access article ]
- Ader, T., M. Chendorain, M. Free, T. Saarno, P. Heikkinen, P.E. Malin, P. Leary, G. Kwiatek, G. Dresen, F. Bluemle, and T. Vuorinen (2019). Design and implementation of a traffic light system for deep geothermal well stimulation in Finland, J. Seismol. DOI: 10.1007/s10950-019-09853-y. [ Article Page ]
- Leonhardt, M., G. Kwiatek, P. Martínez-Garzón, M. Bohnhoff, T. Saarno, P. Heikkinen, and G. Dresen (2021). Seismicity during and after stimulation of a 6.1 km deep enhanced geothermal system in Helsinki, Finland. Solid Earth 12, 581–594, DOI: 10.5194/se-12-581-2021. [ Article Page ] [ Link to associated data publication ]
- Kwiatek, G., P. Martínez-Garzón, J. Davidsen, P. Malin, A. Karjalainen, M. Bohnhoff, and G. Dresen (2022). Limited Earthquake Interaction During a Geothermal Hydraulic Stimulation in Helsinki, Finland, Journal of Geophysical Research: Solid Earth 127, no. 9, e2022JB024354, DOI: 10.1029/2022JB024354. [ Article Page ] [ Download open-access article ] [ Link to associated data publication ]
- Holmgren, J. M., G. Kwiatek, and M. J. Werner (2023). Nonsystematic Rupture Directivity of Geothermal Energy Induced Microseismicity in Helsinki, Finland, Journal of Geophysical Research: Solid Earth 128, no. 3, e2022JB025226, DOI: 10.1029/2022JB025226. [ Article Page ] [ Download open-access article ] [ Link to data publication ]
- Kwiatek, G., I. Grigoratos, and S. Wiemer (2024). Variability of Seismicity Rates and Maximum Magnitude for Adjacent Hydraulic Stimulations. Seismological Research Letters, DOI: 10.1785/0220240043. [ Article Page ]
- Karimpouli, S., G. Kwiatek, P. Martínez-Garzón, D. Caus, L. Wang, G. Dresen, and M. Bohnhoff (2025). Forecasting induced seismicity in enhanced geothermal systems using machine learning: challenges and opportunities. Geophysical Journal International 242, ggaf155, DOI: 10.1093/gji/ggaf155. [ Article Page ] [ Download open-access article ]
Other interesting publications related to the project
- Eulenfeld, T., G. Hillers, T. A. T. Vuorinen, and U. Wegler (2023). Induced Earthquake Source Parameters, Attenuation, and Site Effects From Waveform Envelopes in the Fennoscandian Shield, Journal of Geophysical Research: Solid Earth 128, no. 4, e2022JB025162, doi 10.1029/2022JB025162.
- Hillers, G., T. A. T. Vuorinen, M. R. Uski, J. T. Kortström, P. B. Mäntyniemi, T. Tiira, P. E. Malin, and T. Saarno (2020). The 2018 Geothermal Reservoir Stimulation in Espoo/Helsinki, Southern Finland: Seismic Network Anatomy and Data Features, Seismological Research Letters 91, no. 2A, 770–786, doi 10.1785/0220190253.
- Kim, T., and J.-P. Avouac (2023). Stress-Based and Convolutional Forecasting of Injection-Induced Seismicity: Application to the Otaniemi Geothermal Reservoir Stimulation, Journal of Geophysical Research: Solid Earth 128, no. 4, e2022JB024960, doi 10.1029/2022JB024960.
- Krenz, L., S. Wolf, G. Hillers, A.-A. Gabriel, and M. Bader (2023). Numerical Simulations of Seismoacoustic Nuisance Patterns from an Induced M 1.8 Earthquake in the Helsinki, Southern Finland, Metropolitan Area, The Bulletin of the Seismological Society of America 113, no. 4, 1596–1615, doi 10.1785/0120220225.
- Kukkonen, I. T., P. J. Heikkinen, P. E. Malin, J. Renner, G. Dresen, A. Karjalainen, J. Rytkönen, and J. Solantie (2023). Hydraulic conductivity of the crystalline crust: Insights from hydraulic stimulation and induced seismicity of an enhanced geothermal system pilot reservoir at 6 km depth, Espoo, southern Finland, Geothermics 112, 102743, doi 10.1016/j.geothermics.2023.102743.
- Lamb, O. D., J. M. Lees, P. E. Malin, and T. Saarno (2021). Audible acoustics from low-magnitude fluid-induced earthquakes in Finland, Scientific Reports 11, no. 1, 19206, doi 10.1038/s41598-021-98701-6.
- Rintamäki, A. E. et al. (2021). A Seismic Network to Monitor the 2020 EGS Stimulation in the Espoo/Helsinki Area, Southern Finland, Seismological Research Letters doi 10.1785/0220210195.
- Taylor, G., G. Hillers, and T. A. T. Vuorinen (2021). Using Array-Derived Rotational Motion to Obtain Local Wave Propagation Properties From Earthquakes Induced by the 2018 Geothermal Stimulation in Finland, Geophysical Research Letters 48, no. 6, e2020GL090403, doi 10.1029/2020GL090403.
Other electronic materials
You can also have at the video presentation related to the analysis of St1 seismicity from 2020 stimulation that was presented during 2020 annual meeting of the Seismological Society of America and at the EGU 2020 virtual conference:
The development and operation of the Traffic Light System implemented for the St1 Deep Heat project was presented in another publication by Thomas Ader and co-authors, published in the Journal of Seismology in 2019: